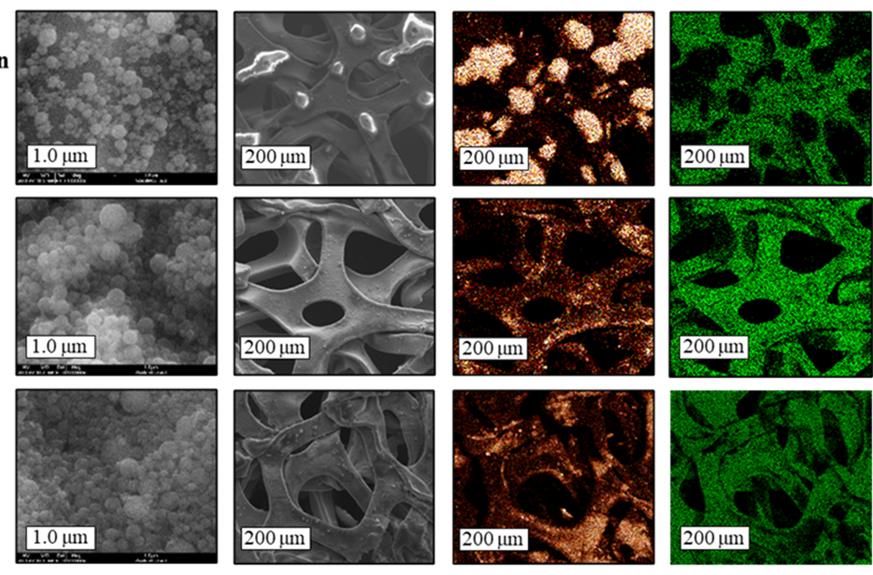
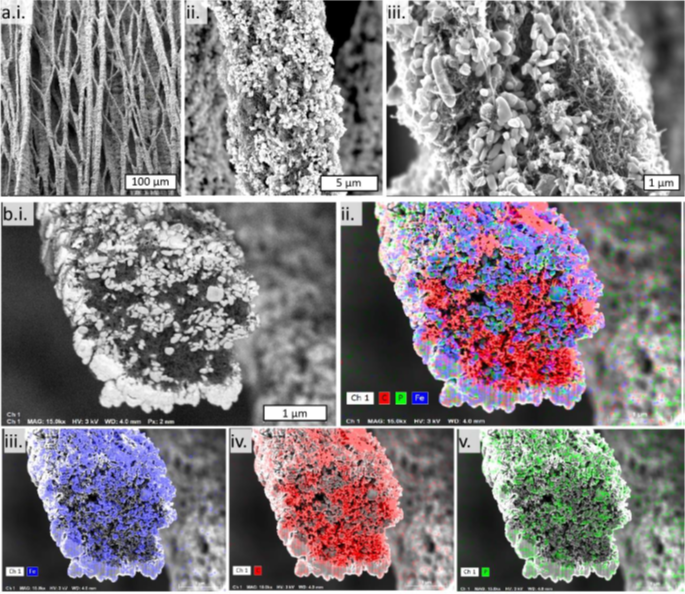
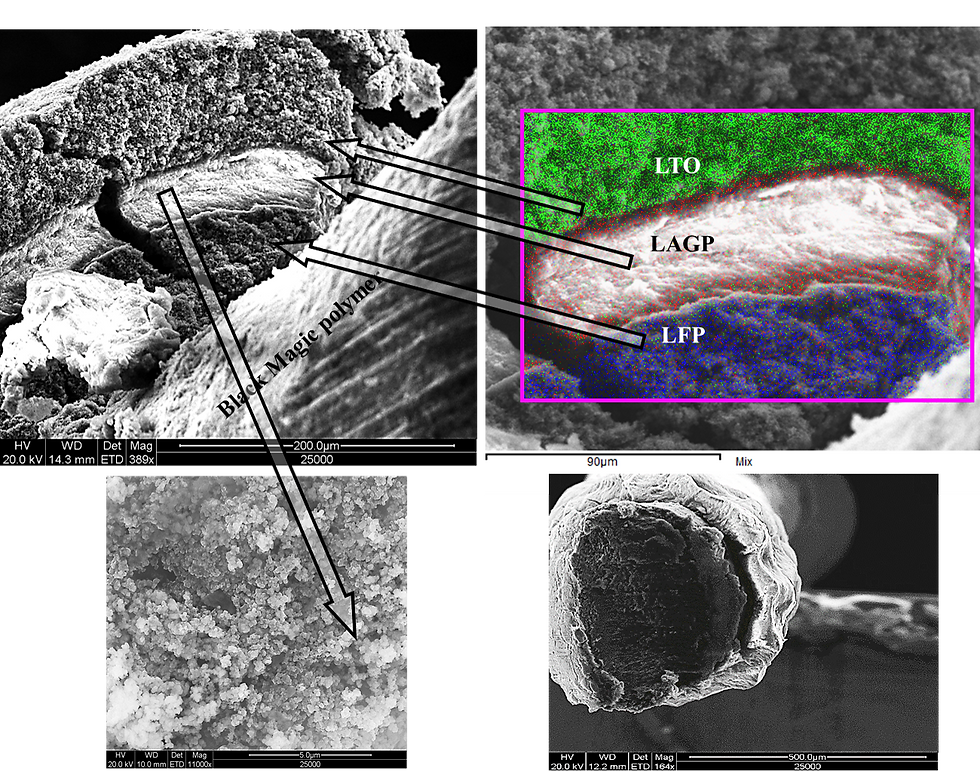
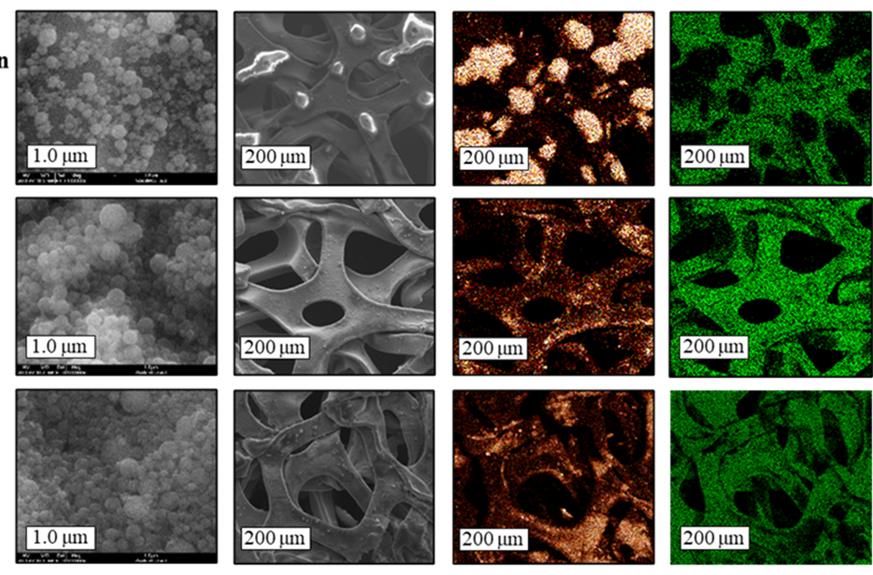
Polymer/Ceramic Interface Barriers - the Fundamental Challenge for Advancing
Composite Solid Electrolytes
Composite solid electrolytes, in which ion-conducting polymers are combined with superionic ceramics, could revolutionize electrochemical-energy-storage devices enabling higher energy density, providing greater stability during operation and enhanced safety. However, the interfacial resistance between the ceramic and polymer phases strongly suppresses the ionic conductivity and presents the main obstacle for these materials.
In the current research, we emphasize the need for a distinct focus on reducing energy barriers to interfacial ion transport and improving cation transference number. Our current focus is on:
1) the development of a fundamental understanding of the parameters controlling interfacial barriers to ion transport between ceramic particles and polymer medium in composite electrolytes;
2) unravelling the influence on ion-transport phenomena in polymer-in-ceramic electrolytes comprising active and inert ceramics and
3) reduction the interfacial barrier to Li + and Na + transport by surface functionalization of ceramic nanoparticles and the creation of an effective conduction path between polymer and ceramic phases.
Owing to high content of ion-conducting ceramics and high t + of polymerized ionic liquids, the fractional contribution of the migrating cationic species to the total ionic conductivity of polymer-in-ceramic electrolytes via a percolation interfacial path, will be close to unity, thus eliminating complications that might arise from emerging concentration gradients during the operation of solid-state batteries. The concept of polymer-in-ceramic electrolytes comprising polymerized ionic liquids may open new vistas in understanding ion dynamics and transport in various composite systems for many fields of science and technology, including energy storage and conversion, chemical separations and water desalination.
Enhancing Electrochemical Performance of Silicon Nanoparticles and Nanowires
Anodes
High-capacity materials are required to address our modern society environmental concerns, ultimately leading to safe and eco-friendly high-energy batteries use in EV and load-levelling applications. We have recently developed composite silicon-based multiphase nanopowders (SiNP) and silicon nanowires (SiNWs)-based anodes. SiNP strategy involves attaching composite silicon nanoparticles to multiwall carbon nanotubes (MWCNT) in order to create a silicon-based active anode material supported by a strong, rigid and high electrically-conducting network.
The method is based on the pyrolysis of the mixture of SiNPs and MWCNTs with carbon precursor. SiNWs are grown on a stainless-steel mesh by a novel catalyst-free chemical vapor deposition process. To improve the mechanical durability and the electrochemical performance of anodes, we coat SiNPs and SiNWs by electroless nickel, followed by annealing at high temperature to receive nickel silicides of different composition. The formation of SiNi alloys has been proved by ESEM-EDS, TEM-EELS, XPS and TOFSIMS methods. Nickel silicide-coated anodes show a much stable electrochemical behavior and high capacity retention. Effect of copper silicides and titanium silicides is under evaluation.
Towards Smart Flexible Batteries
The focus on shifting towards miniaturized products coupled with the booming demand for consumer electronics are some of the key-driving factors behind the flexible-battery market. In the development of innovative power sources, freeing from design limitation along with the synthesis of reliable electrochemical materials with well-tuned features, is considered to be the most important technical prerequisite.
Two approaches for the fabrication of flexible free form-factor batteries, are under development in our group. The first approach utilizes printing techniques. These technologies are still at an early stage, and most currently-printed batteries exploit printed electrodes sandwiching self‐standing commercial polymer membranes, produced by conventional extrusion or papermaking techniques, followed by soaking in non-aqueous liquid electrolytes. We suggest a novel flexible-battery design and already received the initial experimental results on development and characterization of novel 3D printed all-solid-state electrolytes prepared by fused-filament fabrication (FFF). The electrolytes are composed of LiTFSI, polyethylene oxide (PEO), which is a known lithium-ion conductor, and polylactic acid (PLA) for enhanced mechanical properties and high-temperature durability. The flexible all-solid LiTFSI-based electrolyte exhibits bulk ionic conductivity of 3×10 −5 S/cm at 90 C° and 156 ohm/cm^2 resistance of the solid electrolyte interphase (SEI). These results pave the way for a fully printed solid battery, which enables free-form-factor flexible geometries.
The second approach is a unique, single-step method for the preparation of a membrane-electrode assembly. In the low-ionic-strength suspension the migration of both, positively charged particles and free ions (protons), followed by reduction of the latter is most likely to govern the cathodic EPD process. Passivation of the counter electrode (Ni or Al) by dissolved-in acetone oxygen and migration of negatively charged particles control the anodic EPD. In the electrolytic bath with separate electrode partitions, the nanoporous membrane sandwiched between the electrodes serves as the physical barrier to the two-way penetration of particles, the surface charge of which is induced by the adsorption of polyelectrolytes. The applied strong electric field facilitates coagulation of micelles in close proximity to the membrane and precipitation of positively and negatively charged particles on
its opposite sides. This is followed by the formation of a tri-layer membrane electrode battery assembly. Positive and negative battery electrodes (LFP and LTO) on opposite sides of a commercial nanoporous membrane (Celgard 2325) have been recently prepared. The electrodes can be deposited either cathodically or anodically by replacing the interchangeable charging agents, like polyethyleneimine and polyacrylic acid. These polyelectrolytes, when adsorbed on the particles of the active material, serve also as the binders.
The simultaneous EPD can be used for the simple and low-cost manufacturing of a variety of cathode and anode materials on nanoporous polymer- and ceramic ion-conducting membranes for energy storage devices.
In-operando Terahertz Spectroscopy for the study of interfacial phenomena in
batteries
The in-situ characterization of materials and interphases of battery electrodes is of crucial importance for the basic understanding of the complex, simultaneous processes that take place during the operation of secondary batteries. We attempted the use of a Terahertz (THz) time-domain- spectroscopy method for the in-operando characterization of the silicon/electrolyte interfacial phenomena and dynamics in a working lithium-ion battery. Our results show that THz-TDS is able to detect alterations of the SEI under cycling conditions, and more importantly, during rest periods. To the best of our knowledge, this is the first time a spectroscopic method for the characterization of electrodes in lithium-ion batteries provides in-operando monitoring of the possible SEI dissolution in the electrolyte. By on-line monitoring of the THz reflectivity from the electrode-electrolyte interface within a working electrochemical cell, we were able to identify the SEI formation on the surface of the Si electrode during the lithiation process, and its partial dissolution during prolonged delithiation and subsequent rest periods. The presented technique lays the basis for future utilization of terahertz spectroscopy as a uniquely desirable tool in the ever-growing field of in-operando characterization of electrochemical cells. The research is in progress.
Study of 3D Binder-Free Silicide/Silicon Anodes for Lithium-Ion Batteries
Silicon anode materials have attracted much attention as an alternative to the graphite anode in Li-ion batteries since the theoretical capacity of silicon an order of magnitude higher than that of graphite. However, the drastic volume changes of silicon during lithiation/de-lithiation cause breakup of the electrode, electrical isolation of the active material and capacity fade. Binders and conducting agents, while improving adhesion and electrical conductivity, reduce the volumetric capacity of the Si anodes.
We develop and study easy-to-fabricate binder-free 3D silicon anodes with improved performance. The anodes are prepared by combining for the first time three approaches: use of Si nanoparticles, use of porous, high-surface-area metal foam current collector and formation of metal silicide layer in between. The fabrication of 3D anodes includes electrophoretic deposition of silicon nanoparticles (SiNP) on copper, nickel, and titanium foams followed by annealing at different temperatures and time. Analysis of morphology and electrochemical performance of composite 3D silicon/silicide anodes reveals that increased annealing time of SiNPs-deposited on Ni foam results in a thicker Ni3Si2 layer, which leads to the enhanced capacity retention and power capability. At C/10 and C/2 rates the reversible capacity of NMC/3DSi-Ni3Si2 cells was 880 and 530 mAh/g(Si+Silicide), respectively.
Polymer-based LFP/LTO /current collector microfiber-meshes with bi- and interlayered architectures for Li-ion battery
We develop and characterize a free-standing fiber-based mesh cathodes and anodes made of electrospun composite microfibers of lithium iron phosphate (LFP), lithium titanate (LTO) as well as conductive microfibers containing carbon nano-fillers acting as the current collector (CC). Neither the electrodes nor the current collector undergoe post-fabrication treatment or calcination. The meshes are constructed of well-shaped microfibers and exhibit a high porosity, enabling efficient electrolyte penetration and improved electron and ion-transport channels.
Two cathode architectures of the LFP/polymer-based CC meshes were explored: bilayered and interlayered. Both are characterized by a high surface-to-volume ratio. The interlayered structure showed superior electrochemical performance due to enhanced LFP-CC fiber-to-fiber contacts and reduced resistance. Comparative analysis with electrospun LFP on aluminum foil revealed comparable specific capacity but higher polarization in the electrospun LFP/PBCC meshes, attributed to increased internal resistance and limited fiber-to-fiber contacts. However, the electrospun interlayered LFP/CC mesh exhibits significantly higher gravimetric energy density (197 Wh/kg (LFP+PBCC) and 94 Wh/kg (LFP+Al), respectively), offering lightweight and higher-energy-density electrode materials, thus guiding the design of high-performance flexible lithium-ion batteries.
Improving performance of high-voltage Li-ion batteries by composite coatings of the cathode
Over the recent decades, there has been growing interest in researching high-energy-density battery technologies, particularly for electric vehicles (EVs). High-voltage materials, such as Ni-rich lithium nickel manganese cobalt oxide (NMC) layered cathodes, are considered as some of the most effective. However, like other layered-structure cathodes, Ni-rich cathodes are prone to degradation over time. The degradation mechanisms include electrolyte decomposition, structural changes and irreversible phase transitions in the cathode, dissolution of transition metal (TM) cations, oxygen release, and growth of microcracks.
In our research we use a simple and fast electrophoretic deposition (EPD) method to produce electrochemically active coatings as the artificial cathode electrolyte interphase (ART-CEI) for cathode protection. Two types of strong- and poor- ion conducting materials - Li6PS5Cl (LPSC) nanoparticles from the argyrodite family, synthesized in Bar-Ilan University and ball milled LiF have been investigated. The deposited films, in which polymerized ionic liquids (PIL), were used as the binders, had good adhesion to the surface of the cathode without the need for an additional step, such as calcination.
We have found that capacity retention at high charge voltages (4.3 and 4.5 V) is improved due to the ART-CEI. In addition, the electrochemical performance of the coated cathodes shows an enhancement in the high-rate capability and lower resistances of the anode solid electrolyte interphase (SEI), the cathode electrode interphase (CEI), and charge transfer during prolong cycling. The increase in performance is attributed to the suppression of the detrimental side reactions at the cathode-electrolyte interface and modification of electrolyte, while maintaining efficient diffusion of lithium ions through ART-CEI. The mechanisms of protection are thoroughly investigated.